We explore fundamental aspects of quantum gases physics in microgravity and space through theoretical investigations supporting experiments. Operating in these environments, quantum gases at ultracold temperatures exhibit extraordinary behaviors that are challenging to observe on Earth. We support experimental teams using multiple microgravity facilities: the Cold Atom Lab (CAL) onboard the International Space Station (ISS) [1], the MAIUS sounding rocket missions [2], the Einstein Elevator in Hannover [3] and QUANTUS in the Bremen drop tower [4].
Manipulation of Quantum Gases in Microgravity
We develop methods for precise control over quantum gases in microgravity conditions. Fast transport protocols aim to shuttle atomic clouds with remarkable nanometre accuracy employing atom chips [5]. Subsequent delta-kick collimation (DKC) is employed to collimate Bose-Einstein condensates (BEC) and effectively reduce their expansion energy to picokelvin energy scales [5,6]. Our research also delves into optimal control theory for designing fast ramps in transporting Bose-Einstein condensates with atom chips [5]. The combination of these methods allow extended free-fall times for various experiments such as long-time atom interferometry experiments.
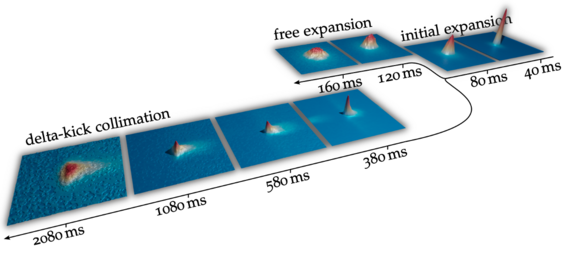
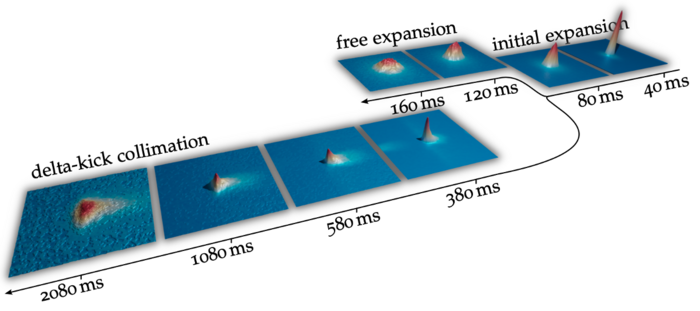
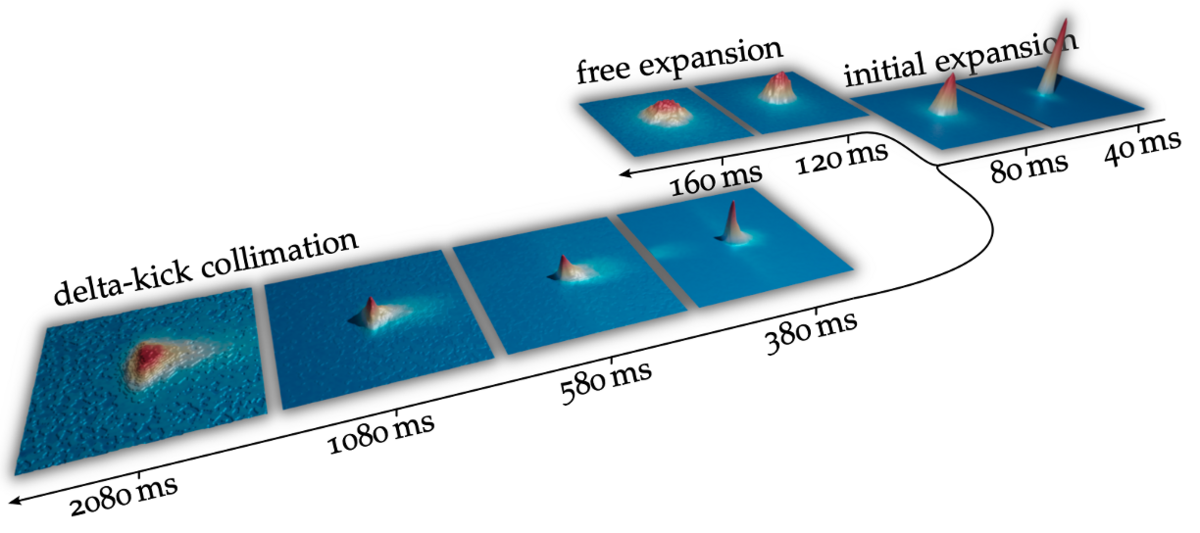
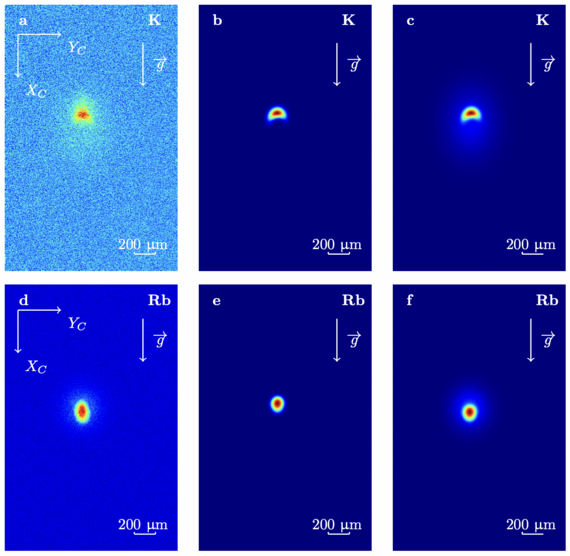
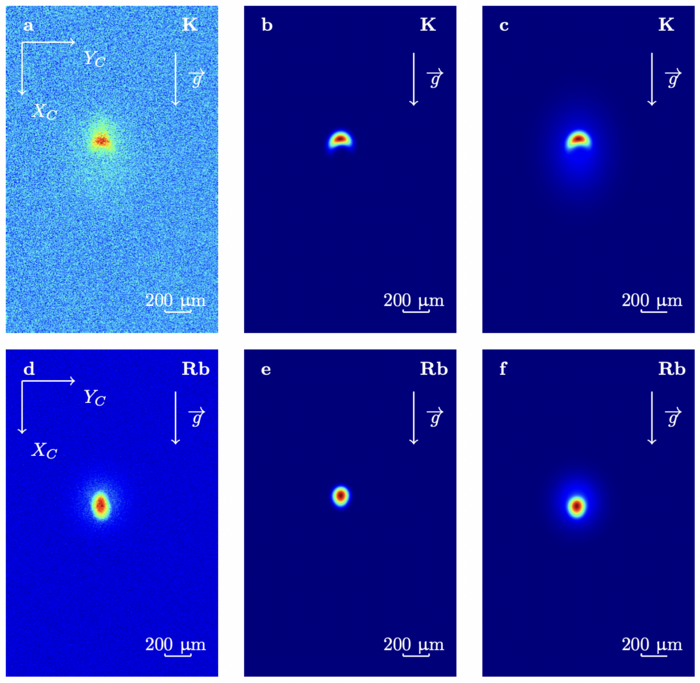
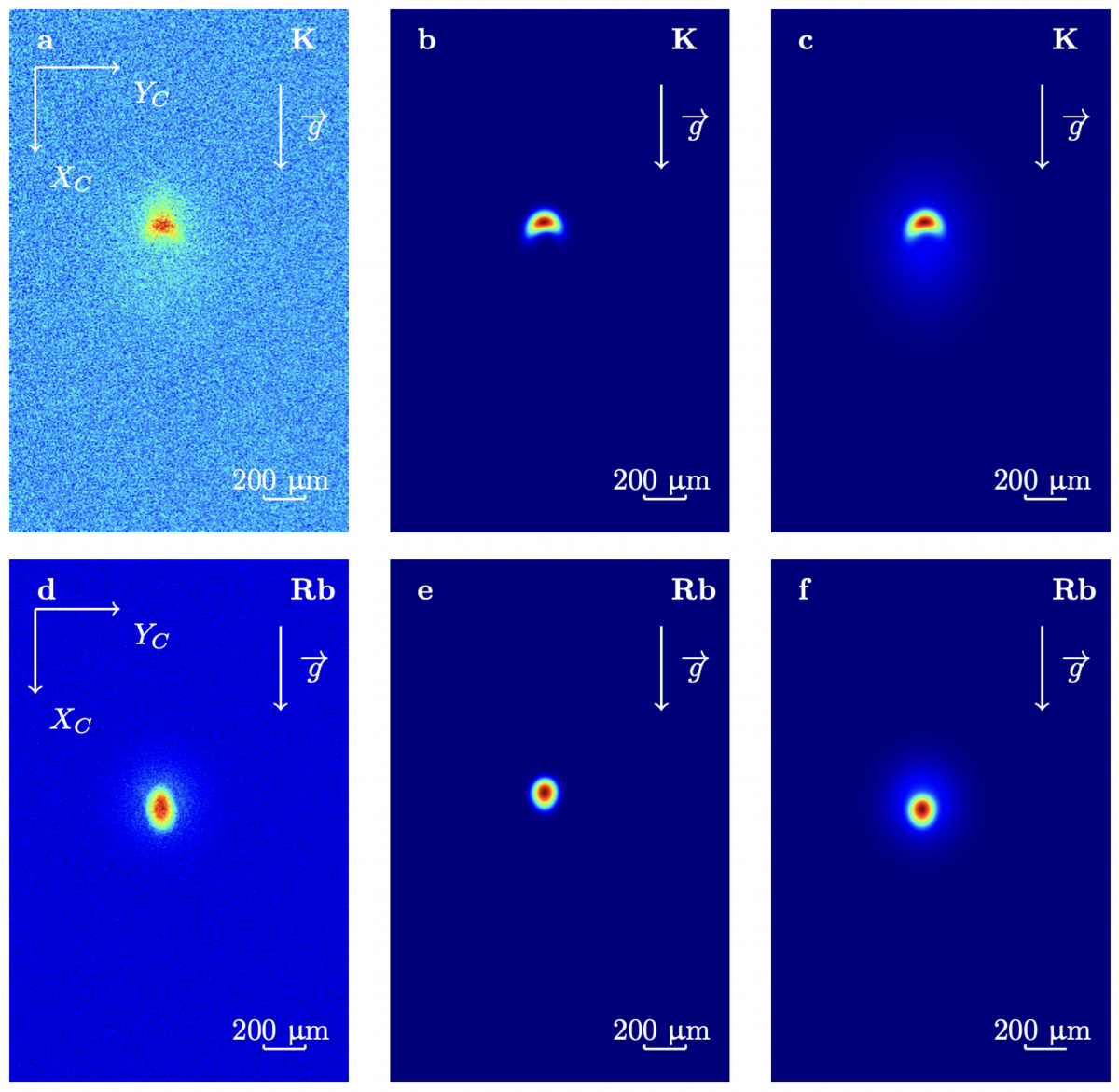
Quantum Mixtures in Microgravity
Our work on quantum mixtures primarily involves simulations of atomic dynamics and interspecies interactions. Achieving a perfect overlap of traps for multiple species of ultracold atoms is notoriously challenging on Earth due to gravity-induced spatial separation. However, in microgravity, this limitation is removed. Our theoretical models and simulations help us understand the dynamics of interacting multi-species quantum gases, particularly in time-dependent potentials. The computational framework, based on a grid-scaling techniques [7], offers efficient numerical solutions to the coupled Gross-Pitaevskii equations governing these systems, making it feasible to describe the long-time behavior relevant to microgravity and space experiments. These methods have been applied to pioneering experiments with interacting 87-Rb and 41-K BECs in MAIUS-B on ground [7] and in Space with CAL [6].
Dual-species BEC Interferometry
Atom interferometry, conducted with multi-species BECs, serves as a powerful tool for testing the Universality of Free Fall (UFF) and probing fundamental physics in the absence of gravitational asymmetry. The first space proof-of-principle experiment was conducted as in the multi-user Cold Atom Lab (CAL) instrument aboard the ISS. Here, a mixture of 87-Rb and 41-K BECs was utilized in a dual atom interferometry scheme [8]. Our simulations played a key role in understanding these findings and executing atom interferometry sequences for various other experiments, such as the first space-based atom interferometry by MAIUS [9].
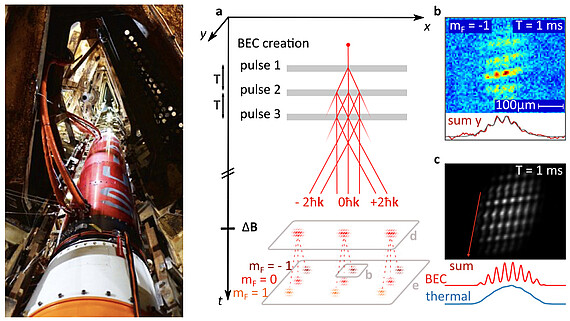
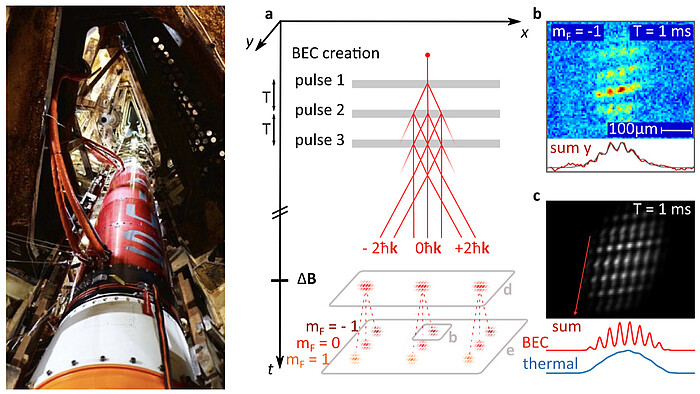
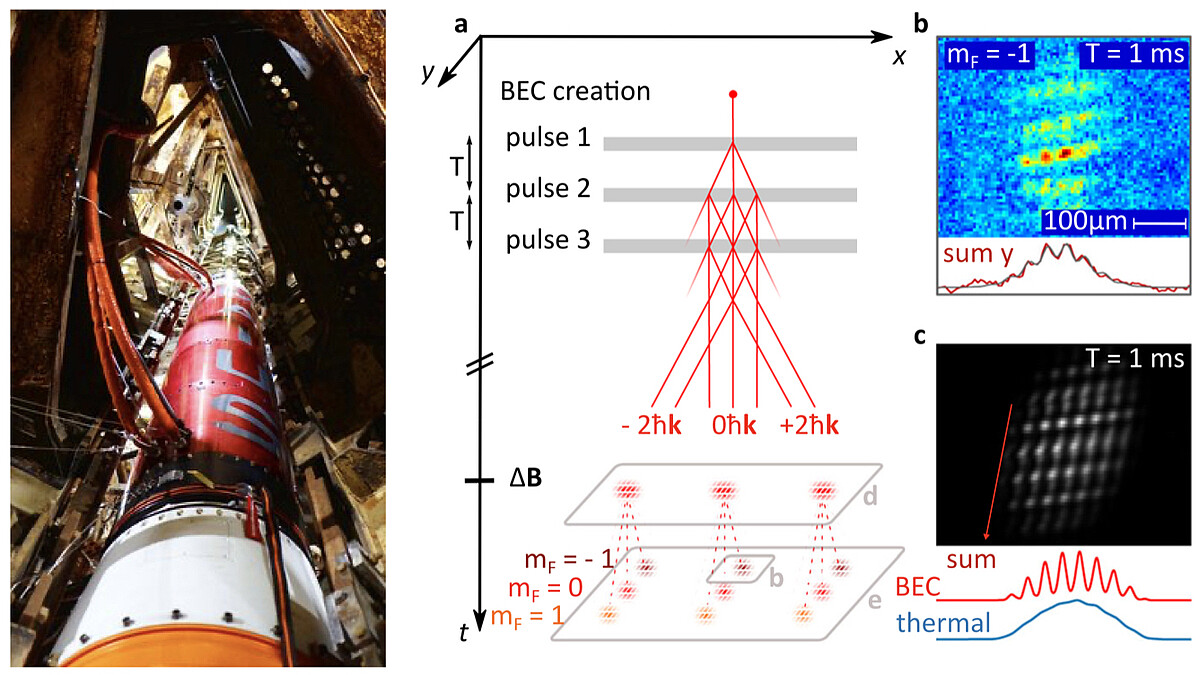
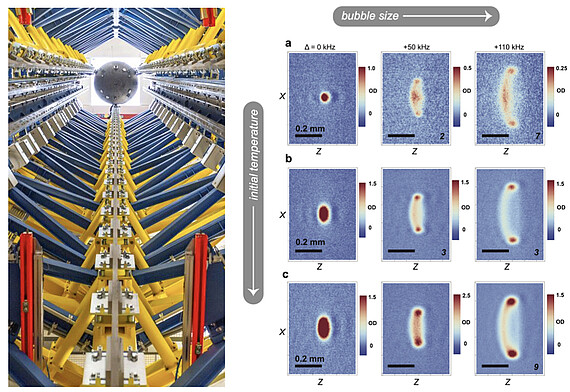
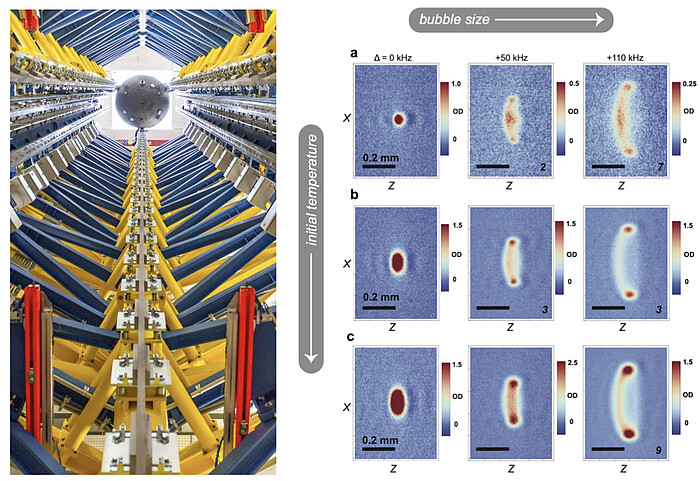
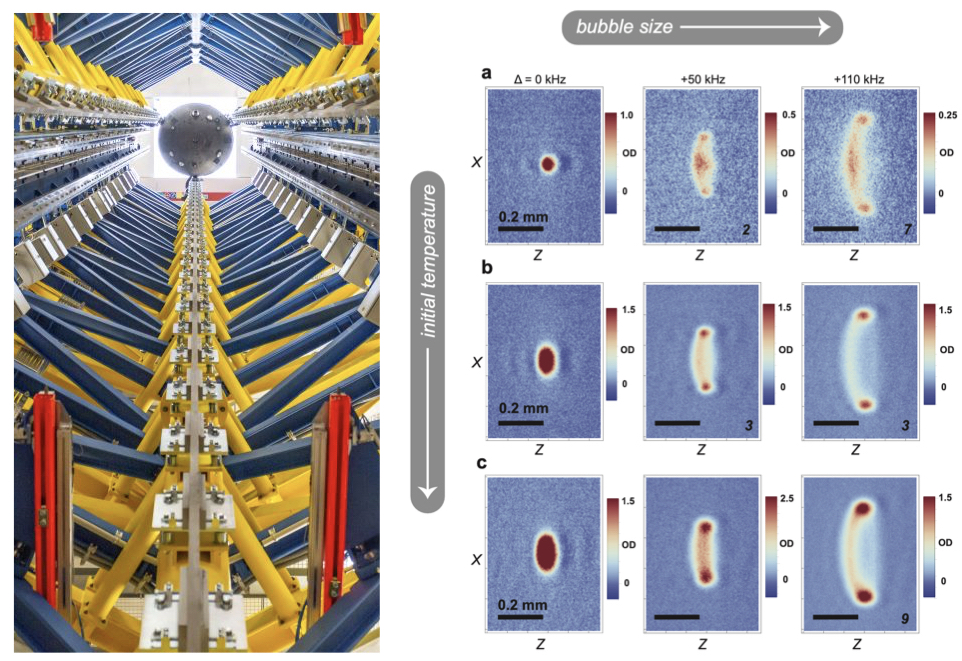
Quantum Bubbles
Quantum gases confined in three-dimensional bubble traps offer novel insights into many-body effects on curved manifolds [10]. Such shell-shaped condensates can for example be created in microgravity by shell-shaped potentials [10] or utilizing multi-species interactions [11]. The first quantum bubble in Space was recently achieved by the Nathan Lundblad consortium in CAL [12]. We started a collaboration with this team to investigate future steps of creating a BEC bubble and more homogeneous shell-shaped potentials with future application such as observing vortices. In parallel, in the DESIRE [13] project using the Einstein Elevator facility at Hitec, Hanover, Germany [3], we aim to extend this work by creating quantum bubbles, allowing for further maturation.
References
[1] Aveline, D.C., Williams, J.R., Elliott, E.R. et al. Observation of Bose–Einstein condensates in an Earth-orbiting research lab. Nature 582, 193–197 (2020).
[2] Becker, D., Lachmann, M.D., Seidel, S.T. et al. Space-borne Bose–Einstein condensation for precision interferometry. Nature 562, 391–395 (2018).
[3] Einstein Elevator source.
[4] Kulas, S., Vogt, C., Resch, A. et al. Miniaturized Lab System for Future Cold Atom Experiments in Microgravity. Microgravity Sci. Technol. 29, 37–48 (2017).
[5] Gaaloul, N., Meister, M., Corgier, R. et al. A space-based quantum gas laboratory at picokelvin energy scales. Nat Commun 13, 7889 (2022).
[6] Christian Deppner, et. al. Collective-Mode Enhanced Matter-Wave Optics. Phys. Rev. Lett. 127, 100401.
[7] Annie Pichery, et. al. Efficient numerical description of the dynamics of interacting multispecies quantum gases. arXiv preprint (2023).
[8] Ethan Elliot, et. al. Quantum Gas Mixtures and Dual-Species Atom Interferometry in Space. arXiv preprint (2023).
[9] Lachmann, M.D., Ahlers, H., Becker, D. et al. Ultracold atom interferometry in space. Nat Commun 12, 1317 (2021).
[10] Lundblad, N., Carollo, R.A., Lannert, C. et al. Shell potentials for microgravity Bose–Einstein condensates. npj Microgravity 5, 30 (2019).[11] A. Wolf, et. al. Shell-shaped Bose-Einstein condensates based on dual-species mixtures.
[12] Carollo, R.A., Aveline, D.C., Rhyno, B. et al. Observation of ultracold atomic bubbles in orbital microgravity. Nature 606, 281–286 (2022).
[13] DESIRE
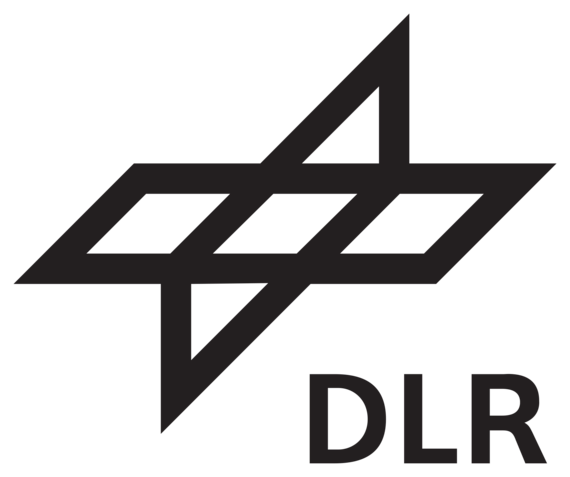
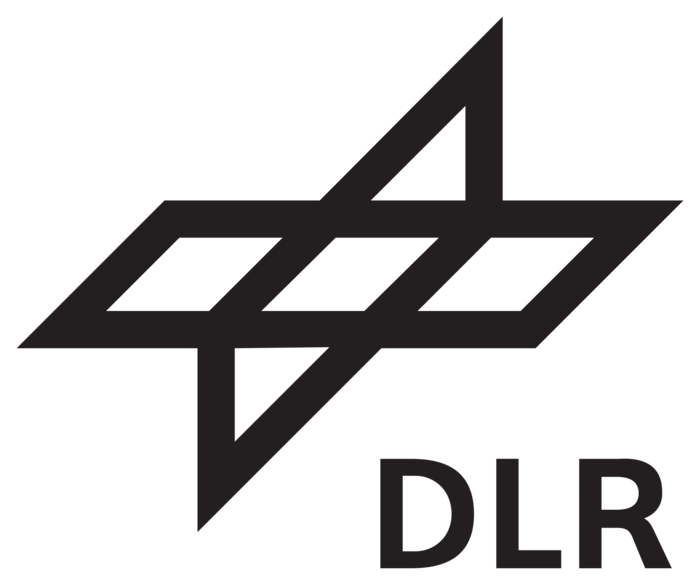
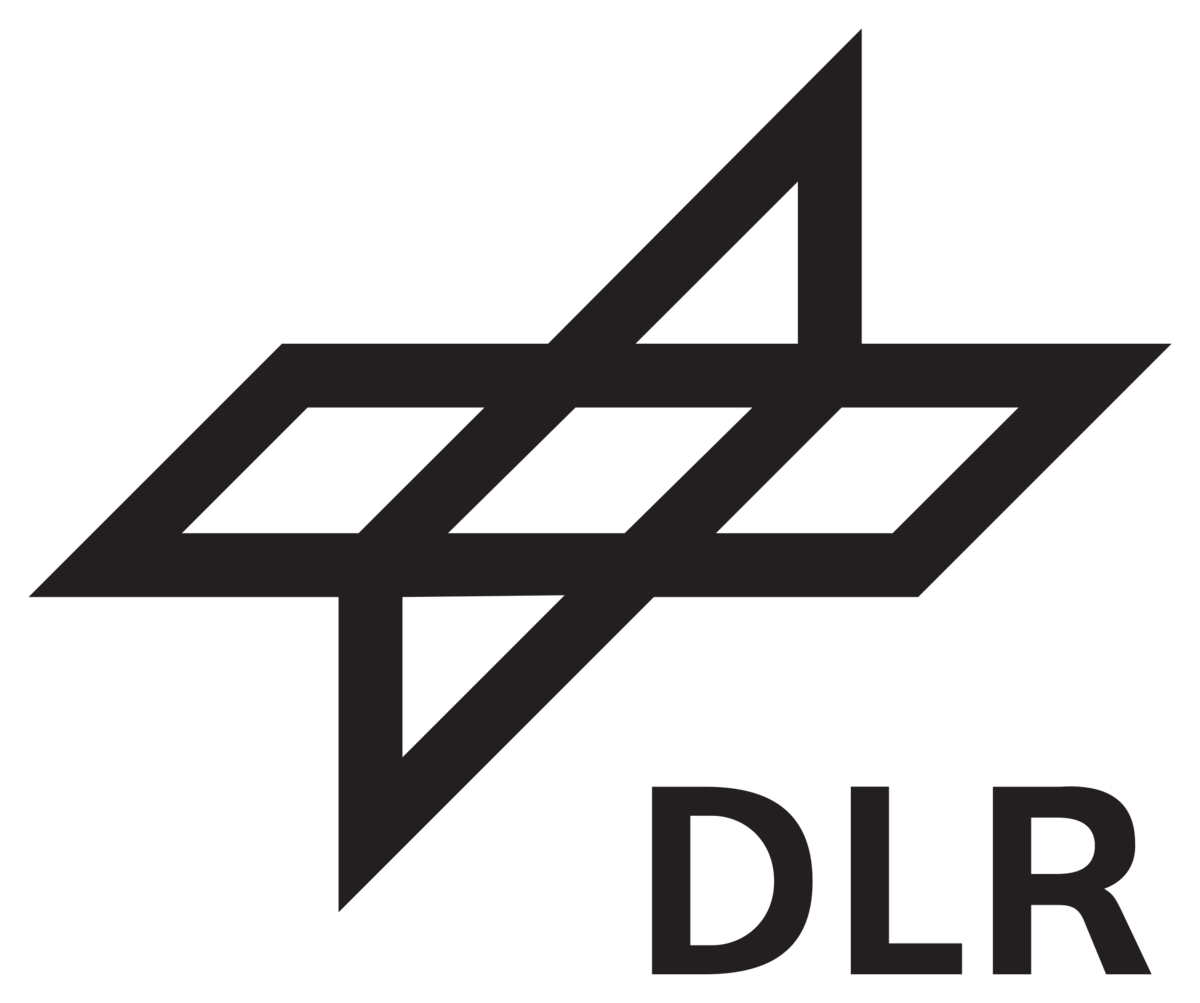
This work is funded by the German Space Agency (DLR) with funds provided by the German Federal Ministry of Economic Affairs and Energy (BMWi) due to an enactment of the German Bundestag under Grant No. 50WM2245A (CAL-II), 50WM2263A (CARIOQA-GE) and 50WM2253A (AI-quadrat).
This work is also supported by the "ADI 2022" project funded by the IDEX Paris-Saclay, ANR-11- IDEX-0003-02