Virtual Lab
Our theoretical and computational research concerns multi-scale and multi-physics light matter interaction with a focus on generation and application of few- and single cycle pulses. This includes modern nonlinear optics, ultrashort pulse propagation in different media such as gases or condensed matter, generation of new frequencies, and strong field phenomena. The investigations comprise the theoretical analysis of the fundamental effects, modeling, and development of adequate numerical methods. High-performance computing and rigorous multi-dimensional simulation are used for realistic descriptions of the experiments. Besides supporting and optimizing the experimental investigations, a strong focus is set on developing new concepts.
PhoenixD
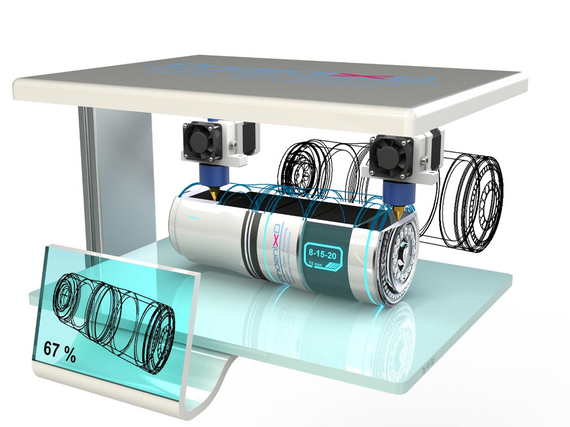

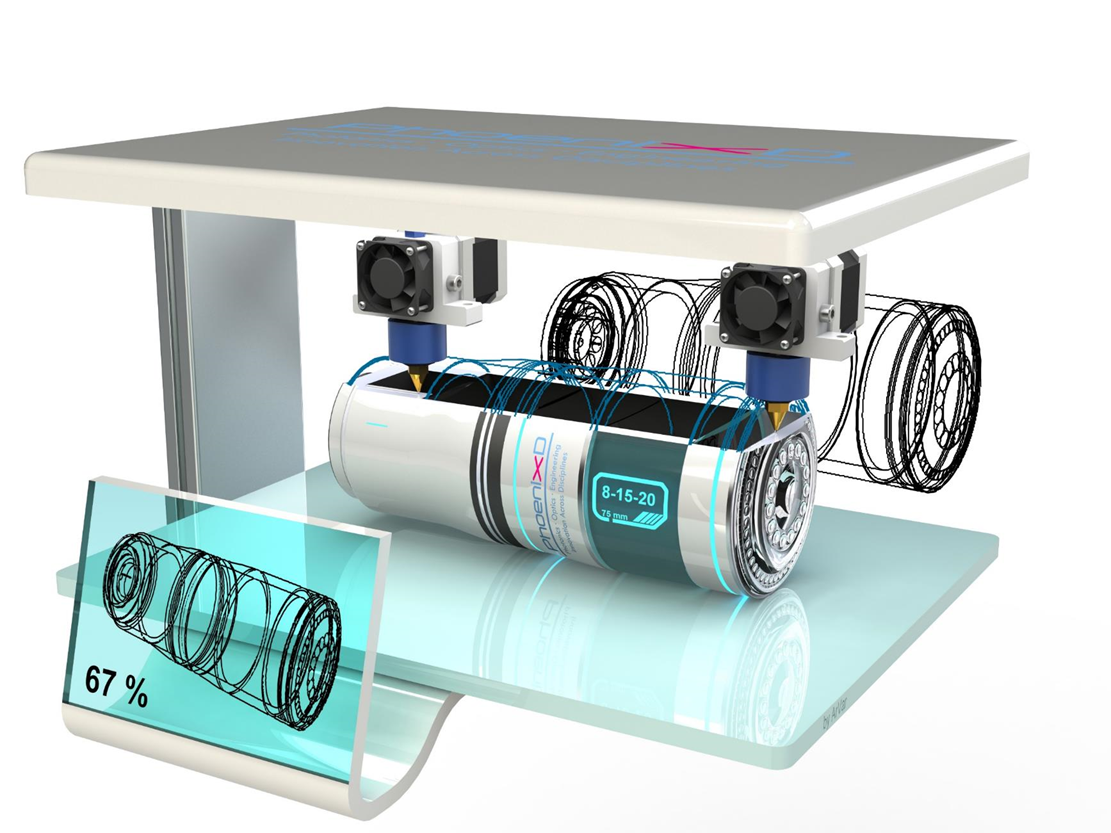
PhoenixD aims to initiate a change of paradigm in optics from large and complex free space high performance optical setups to smart, compact, and comprehensive devices manufactured in one integrated production grid. This approach has the potential for a technological revolution similar to the introduction of integrated electrical circuits.
Spatio-temporal propagation of light pulses through χ(2) media
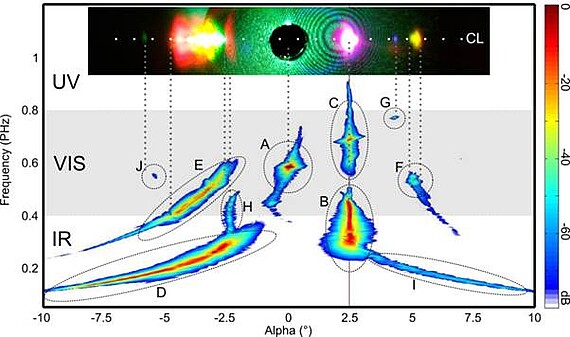
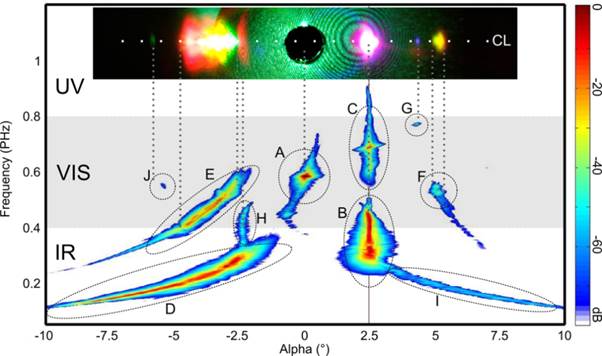
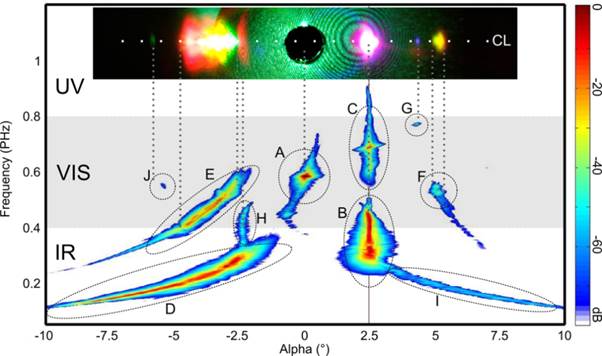
The subject of this project is the investigation of the complex spatiotemporal dynamics occurring during the propagation of broadband pulses in collinear or non-collinear configuration through a nonlinear crystal. The comprehensive analysis of different state-of-the-art OPAs, aided by the use of our (2+1) dimensional simulation model gains knowledge about the interplay of the different intended and parasitic spatiotemporal effects during the propagation (see in the figure multiple colors phase-matched in a green-pumped BBO crystal). The deep insights achieved by these studies helps developing of future OPA and OPO systems based on ultra-broadband non-collinear phase-matching concepts.
Pulse propagation
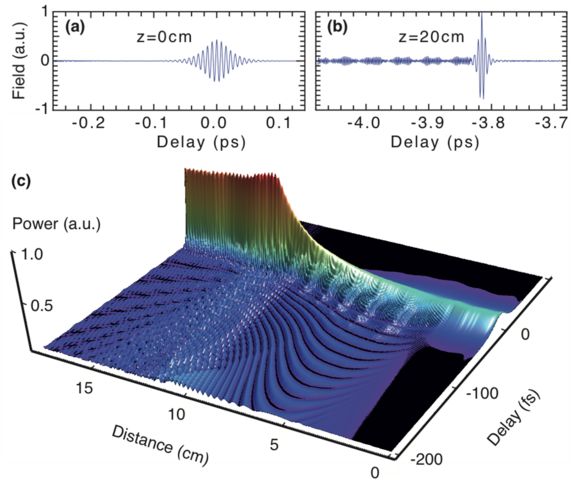
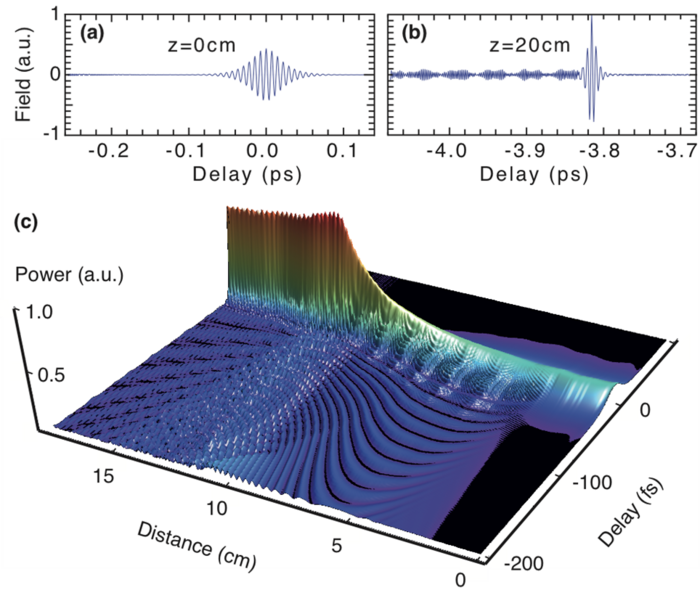
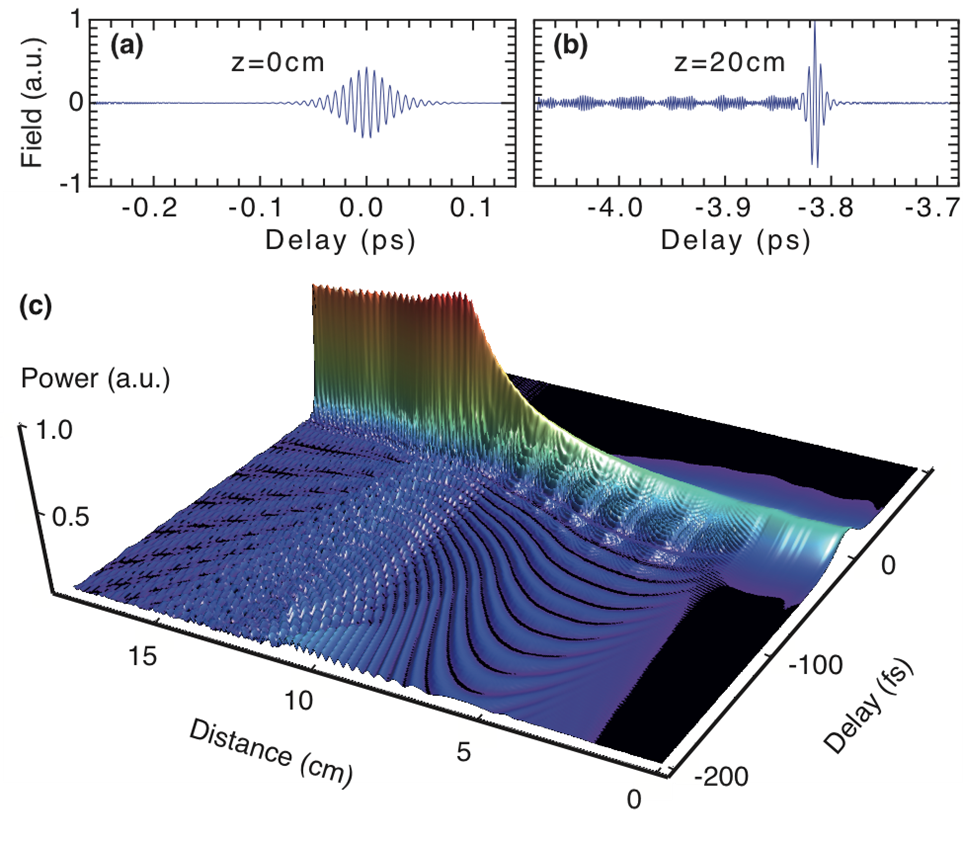
Propagation in dispersive nonlinear media of intense ultrashort pulses, especially in the few-cycle regime, requires modeling beyond the usual approximations, as well as numerical schemes with high accurancy. We develop and use different one- and multi-dimensional propagation models and schemes, to descripe propagation phenomena in fibers, fiber-lasers, fluid jets, or silicon waveguides.
A special focus is set on the generation of new frequency components and coherent octave spanning spectra, known as supercontinuum generation. Besides description of the observed phenomena, the investigations aim to provide new methods and concepts to achieve few-cycle pulses over wide ranges in the spectrum.
Light-matter interaction in strong fields and filamentation
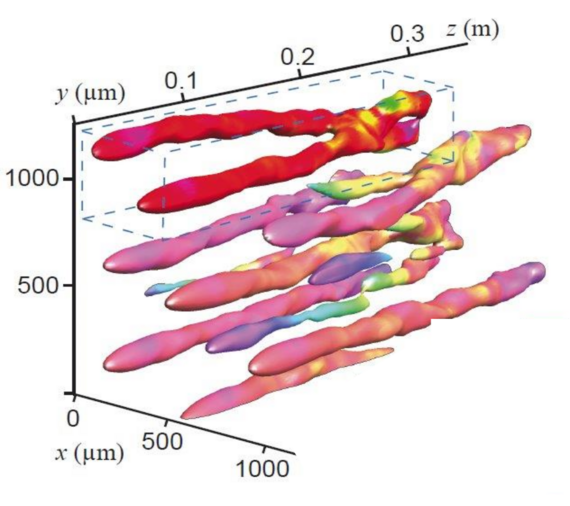
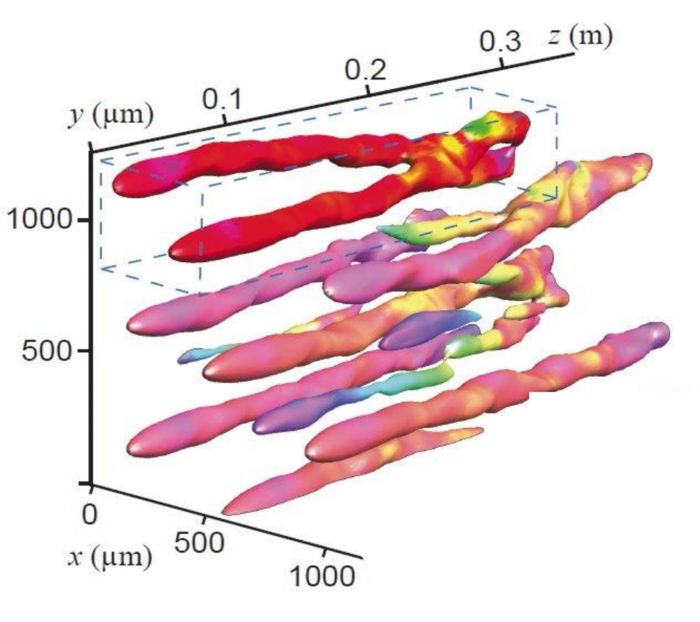
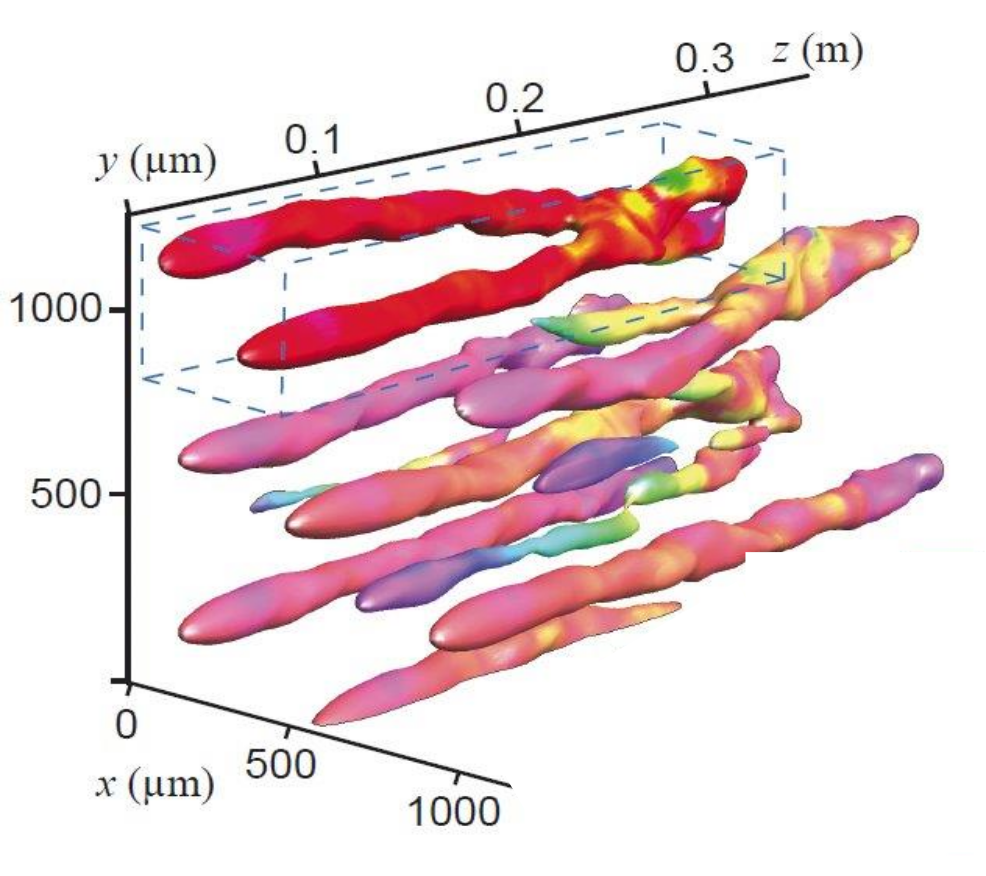
One of the important direction of our group is to study interaction of strong short optical pulses with matter. It is possible nowadays to make short femtosecond pulses where the field strength is comparable to the one of the intra-atomic electric field. Such strong fields can throw out electrons from atoms. This ionization process is one of the fastest studied in labor and takes place on the attosecond time scala. We study the details of this process as well as its applications to generate new frequencies.
In particular, in strong tailored two-color optical fields it is possible to generate efficiently ultrashort pulses of very low frequency, hundred times smaller than the pump one, namely in terahertz (THz) range. This is one of the methods allowing to effectively close so called "THz gap", as this frequency range has been named because of difficulty to produce efficiently tunable THz pulses both with electronic and optical means.
Another remarkable interaction process of intense ultrashort laser pulses with condensed matter and gases under extreme conditions leads to the formation of plasma channels allowing self-guiding over unexpected long distances. This so called filamentation results from a complex interplay of a high number of linear and nonlinear effects and different scenarios of filamentation can be observed. Fully space-time resolved simulations are required to get insight into the details of the resulting dynamics and reveal the impact of the different involved physical mechanisms. This is especially crucial for our direct applications of filamentation given by supercontiuum generation, self-compression, or high-harmonic generation.
Hymnos
Numerical approaches for the description and modeling of light fields in optical media can profit significantly from modern trends in computer technology. In particular, massive parallelization using graphic cards (GPUs) enables new approaches to efficient simulation and offers at relatively moderate cost large computing power and high performance which so far could only be achieved using computer clusters. Also, using current tools a continuous modeling and simulation of light-matter interaction including thermal and mechanical effects is often not possible. This demands for the development of new tools allowing for comprehensive theoretical description of such systems: hybrid optical simulation. In a new joint research project at the Hannover Centre for Optical Technologies (HOT) funded by the VW Stiftung within the Nieders. Vorab program (Grant ZN 3061) we aim to develop novel simulation tools by combining optical, mechanical (acoustic) and thermal simulation concepts. The ultimate goal is to lay the foundations for a deeper understanding of light-matter interaction in complex optical systems. For this purpose, the we investigate dynamic interaction of light fields in various material systems such as dielectrics, semiconductors, fluids, and biological tissue. The innovative aspect of our approach is to include the material response, e.g. thermal effects, in the description of the light propagation.
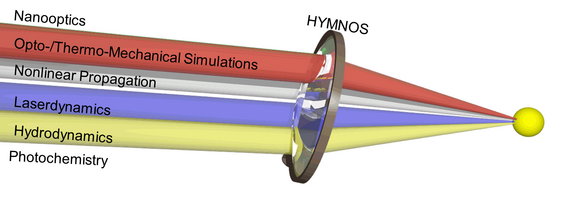
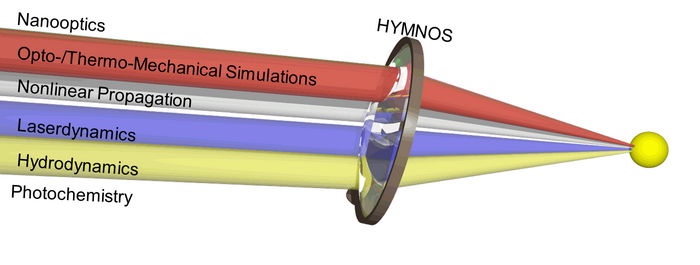
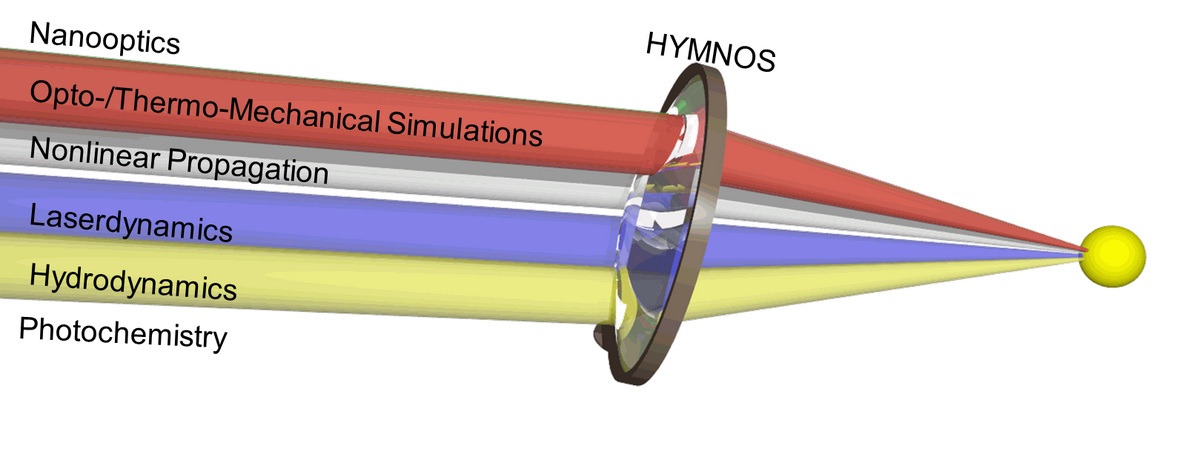